LRF is a device used to measure precise distances. Most LRFs operate on the principle of sending a narrow laser pulse towards an object and measuring the flight time. A receiver then detects the reflection of the light and accurately calculates the distance based on the time it takes for the light to return. When compared to ultrasonic or radio and microwave frequency devices (radar), the main advantage of laser distance measurement techniques is their high resolution. LRFs often include imaging devices that allow the user to precisely direct the laser beam to the object of interest. This way, the device can be spatially matched with the object of interest.
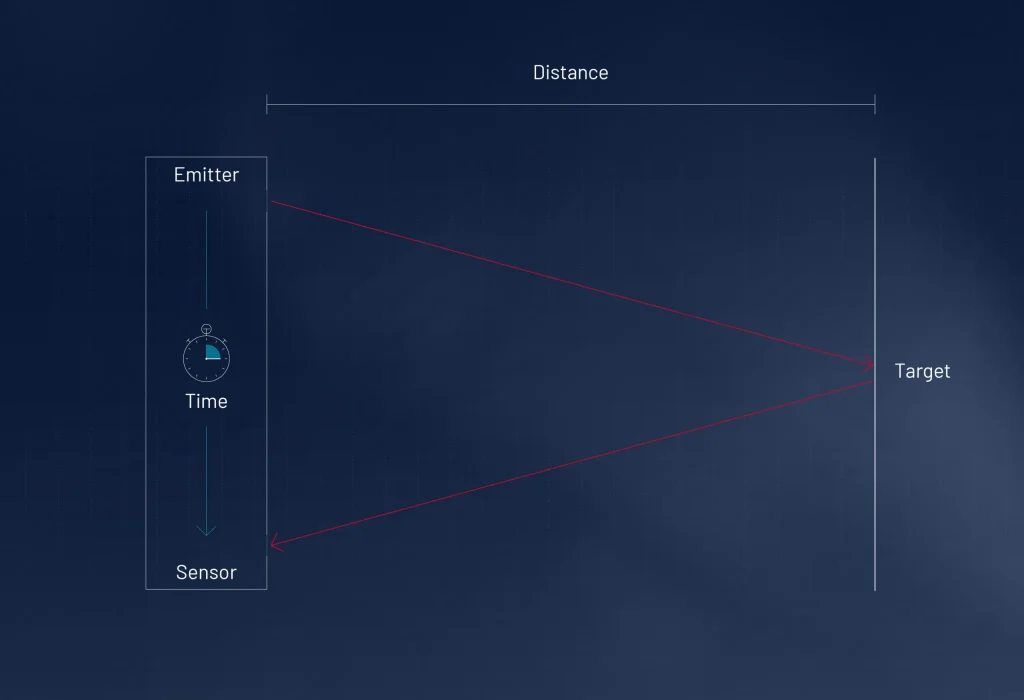
The Basis of Laser Distance Measurement
For long-distance measurements, the most commonly used system is the laser echo (ToF) system. The key factors for flight time are to provide narrow and high-power pulses to maintain accuracy and a high count rate. Time-of-flight laser rangefinders use time measurement as the source of distance measurement. Advances in semiconductor lasers have led to widespread use of semiconductor lasers instead of Q-switching methods.
In LRFs, achievable spatial resolution is limited by pulse duration and/or the speed of the photodetector. Conversely, since there emerges a need for the sensor and the optics collecting light to grow exponentially for signals to be better detected, size and weight properties become especially significant. For signals received at low levels, particularly the use of sensitive photodetectors is highlighted (see also: APD).
Laser beamcan go travel very far under ideal conditions, without changing much. However, its power diminishes due to collisions with impurities in the air. Therefore, all LRFs have a limited range of measurement. The range of measurement depends on many parameters such as; the power of the laser beam, sensor’s sensitivity, and laser beam frequency. In order to achieve the maximum measurement range, the distance to objects with large surface areas like houses and large rocks should be measured. These targets are called “beamfilling targets.” Beamfilling targets are ones that have the reflecting area for all the laser beam spread across certain distances.
Frequently, devices that will operate laser security class 1 are designed in LRF. Thereby, extra precautions for eye safety are no longer required. However, this situation may limit the optic power sent to the target, hence the detecting abilities significantly. The most common method of protection is using a light with longer wavelenght than that of near-infrared. The most commonly encountered wavelengths in laser distance measurements are 905 nm and 1550 nm. LRFs operating at 1550 nm wavelength are safe for the eyes* and are not detectable by traditional vision intensifying night vision goggles. Additionally, they allow for the measurement of longer distances because they are less affected by atmospheric conditions.
In ToF-based systems, the maximum range is dependant on many factors such as; the power of the laser beam, quality of beam, transmitter and receiver optics, choice of the detector, signal processing, etc. The net effect in these types of systems is determined by the detection unit in which all optics are.
Fibers can create a laser beam near the limits of an almost perfect light source. The light spread from the fibers displays a better optical performance compared to the diode lasers, since they can focus and parallelize efficiently. In transmitter optics where a medium like fiber is used, a quite narrow beam can be achieved with a rather small optic (ex. 0.5 mrad beam from 5 mm optical aperture).
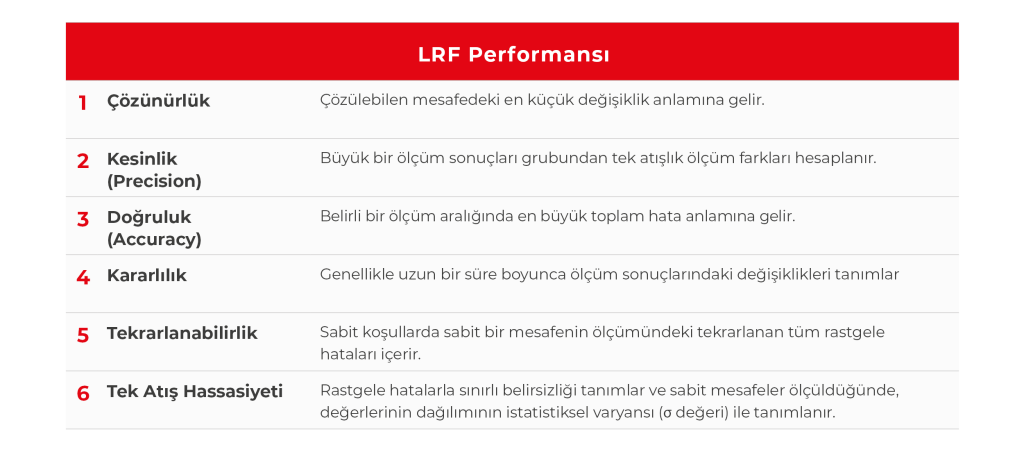
Problems in Laser Distance Measuring and Their Solutions
Beam divergence in long proximities may lead to a significantly increasing point size on the object and atmospheric deteriorations tend to enhance this complication. An increase of the dot size on the object, especially for small objects, can reduce the received signal strength, and may cause distortions on the neighboring objects due to the light dispersed. The greatest advantage of using fiber laser instead of standard laser diode is achieving intense light in smaller scales. In field applications, range performance is largely dependent on beam size and divergence. If the signal transmitted to the target reflects from different environments than the target itself, there may be a decrease in target detection performance. Laser rangefinders may face additional challenges such as the reflection or interference from other objects in the surroundings, not just the object aimed at by the user, or interference like jamming (in military applications).
Features and Functions of Laser Rangefinders
In today’s technology, multiple pulses are used instead of a single pulse for more accurate target measurements. The primary advantage of multiple pulse distance measurement is the reduction of the impact of small vibrations on targeting accuracy in portable systems. The process of hitting targets with laser light can be greatly enhanced using multiple pulses. Multiple pulses can be used continuously, improving performance in target tracking applications. One of the prominent advantages of multiple pulse systems is the ability to adjust system performance (pulse energy, in simpler terms) based on the signal received from pulses. This capability enables consistent detection of targets at various ranges with the same level of accuracy.
Laser pulses typically directed towards a target can reflect from multiple objects and generate signals at the receiver. Algorithms developed to process these signals effectively enable laser rangefinders (LRFs) to distinguish between multiple targets based on distance, thereby allowing them to detect multiple targets.
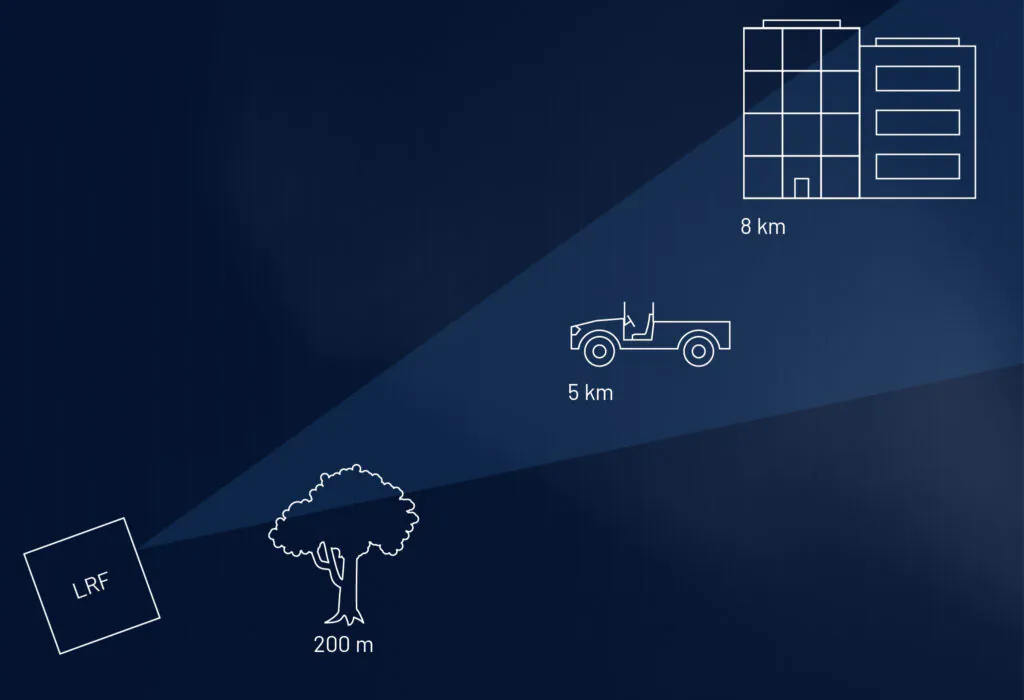
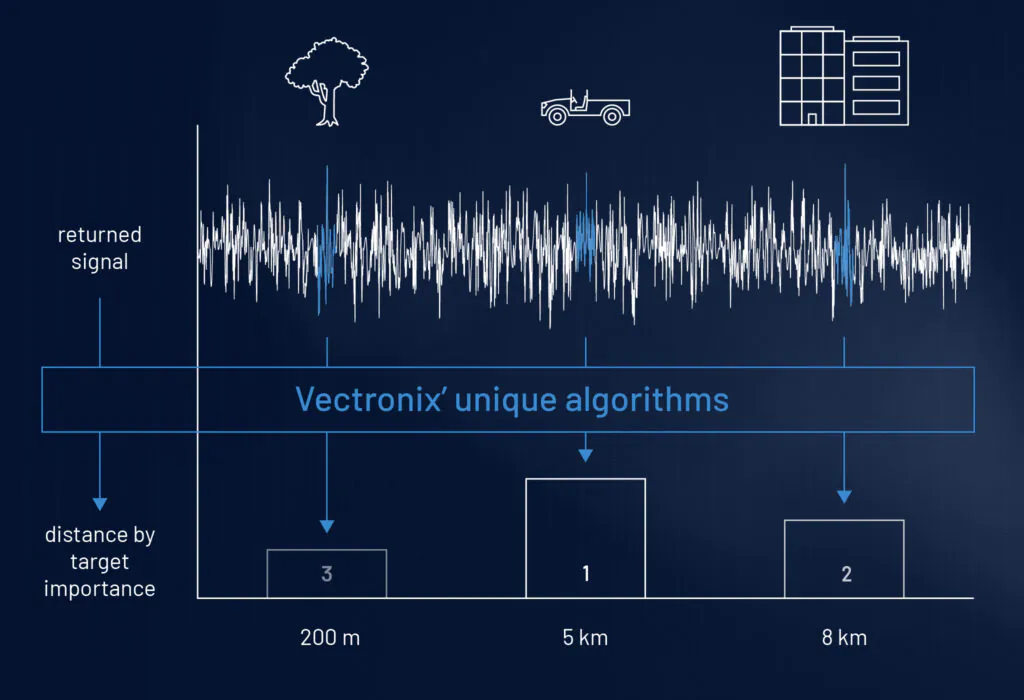
The use of coaxial laser pointers allows laser light directed at targeted objects to be visualized using simple CCD/CMOS-based cameras. The main advantage of easily visualizing targeted objects is the simplification of alignment processes. Coaxial laser technology enables both 1550 nm laser and near-infrared laser to propagate from the same aperture, eliminating the need for any alignment requirement and facilitating the alignment of the LRF with an imager. Despite the perception of low range performance for near-infrared lasers, distances of kilometers can be achieved, especially with high power levels and suitable environmental conditions.
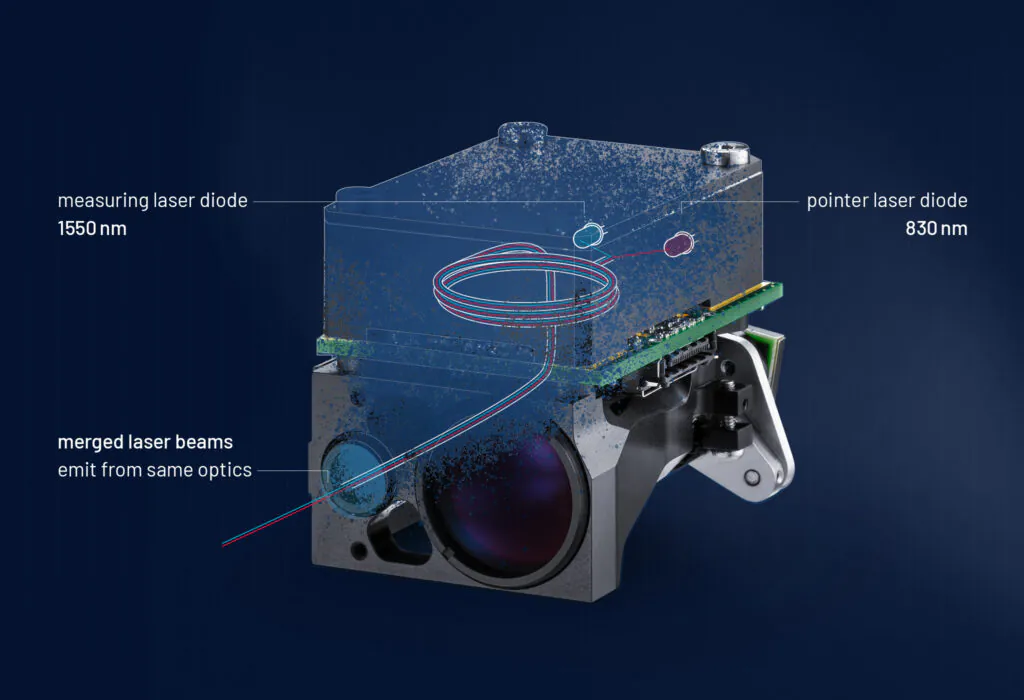
Detecting objects flying at certain speeds like unmanned aircrafts using distance measuring techniques like LRF is quite a challenging application. A laser beam that spreads across a wide area is needed for these types of applications. In this day and age, laser rangefinders (LRFs) with repetition rates around 20 Hz are capable of detecting objects flying at speeds of 50-70 km/h from distances of 1 km (target area: 0.22 m * 0.22 m).
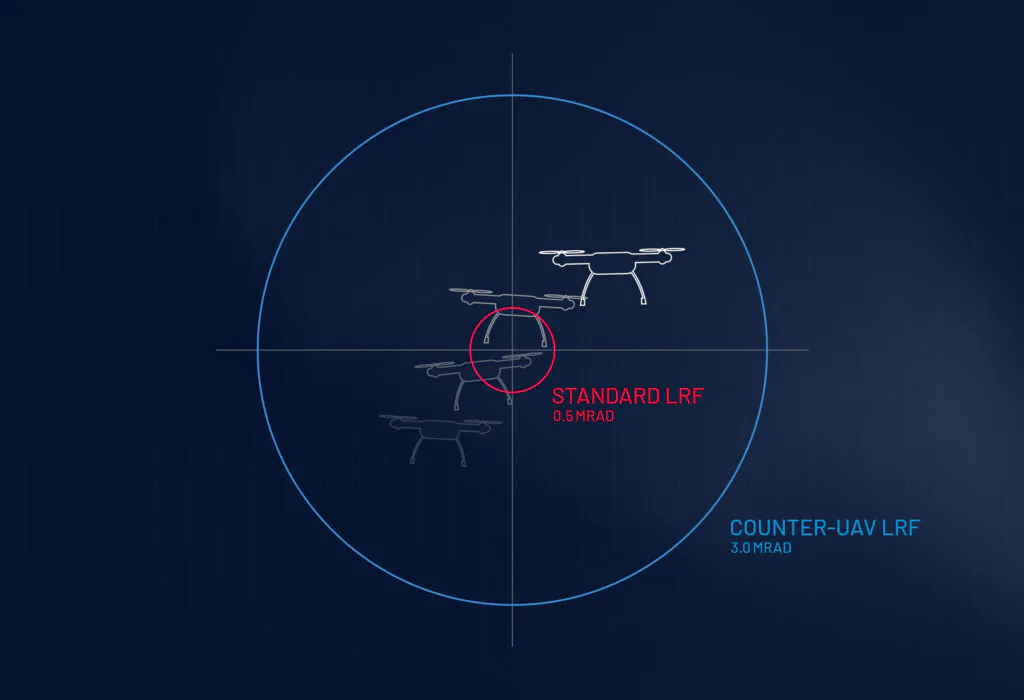
Alternative Distance Measurement Techniques: Advantages and Disadvantages of LRF
In addition to laser distance measurement, there are many techniques for determining distance. RADAR distance finding operates in a similar manner to laser ranging. The only difference is the transmission of a radio signal pulse instead of a focused laser beam. RADAR is more suitable for determining the distance and speed of large objects like aircraft and ships in open areas since it spreads over a wide area and operates at long wavelengths.
The common factor between gigahertz-range RF waves and optical methods is that angular resolution depends on wavelength. As the wavelength decreases, beam divergence decreases. Optical methods are superior when high resolution is required in both horizontal and vertical dimensions. Optical methods provide the best resolution and accuracy in distance measurement.
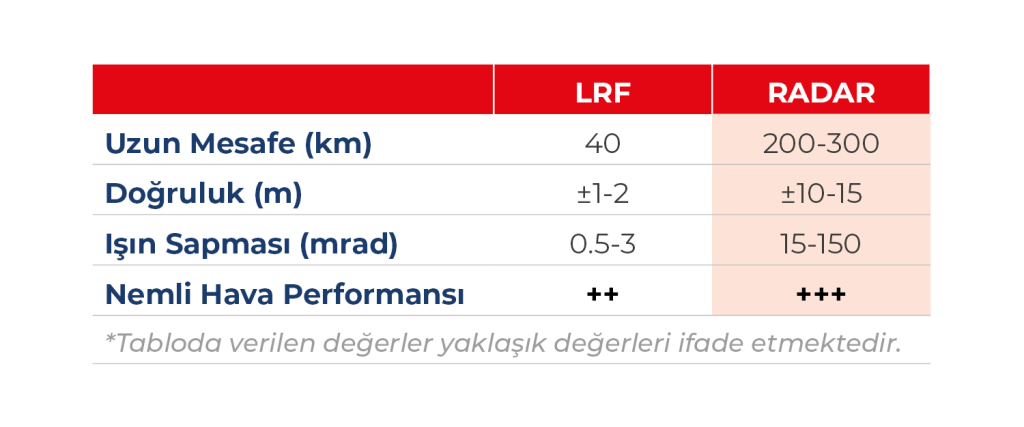
Usage Areas of LRF
• Handheld Devices
• Gimbals
• Fire Control Systems
• Distant Weapon Stations
• Observation and Surveillance Systems
• Coast Guard and Border Protection
Key Questions in LRF Usage
• Visibility/Visibility of the Observer
• Test Target (Size and Projection)
• Weather Conditions
• Platform Information
LRF Key Parameters
• Maximum Range
• Wavelenght
• Beam Divergence
• Distance Measuring Accuracy
• Measurement Repetition Frequency
• Multiple Target Detection
• Eye Safety